Let’s be real: the future doesn’t look like flying cars or silver jumpsuits—it looks like robots the size of bugs doing surgeries inside your body while you nap. Yep, science fiction forgot to tell us the coolest tech would be almost invisible. Over at MIT, researchers have been cooking up an entire sci-fi universe you can literally swallow. We’re talking bug-sized bots that crawl through your guts, swim through blood vessels, and whisper to each other in ultrasound like they’re plotting something (don’t worry—they’re on your side).
Why go so small? Because when it comes to your body, subtle beats scalpel. These tiny machines can reach places a surgeon’s hand never could, treat problems before you even feel them, and vanish without a trace. It’s like having an army of microscopic interns doing diagnostics, repairs, and deliveries inside you 24/7. And the craziest part? This isn’t some distant Jetsons-era dream. These inventions already exist, and they’re getting weirder, smarter, and more life-saving by the day. So let’s dive into 12 mind-blowingly small MIT creations that prove the next big thing in medicine… is basically microscopic.
1. Soft-Muscle-Powered Flier
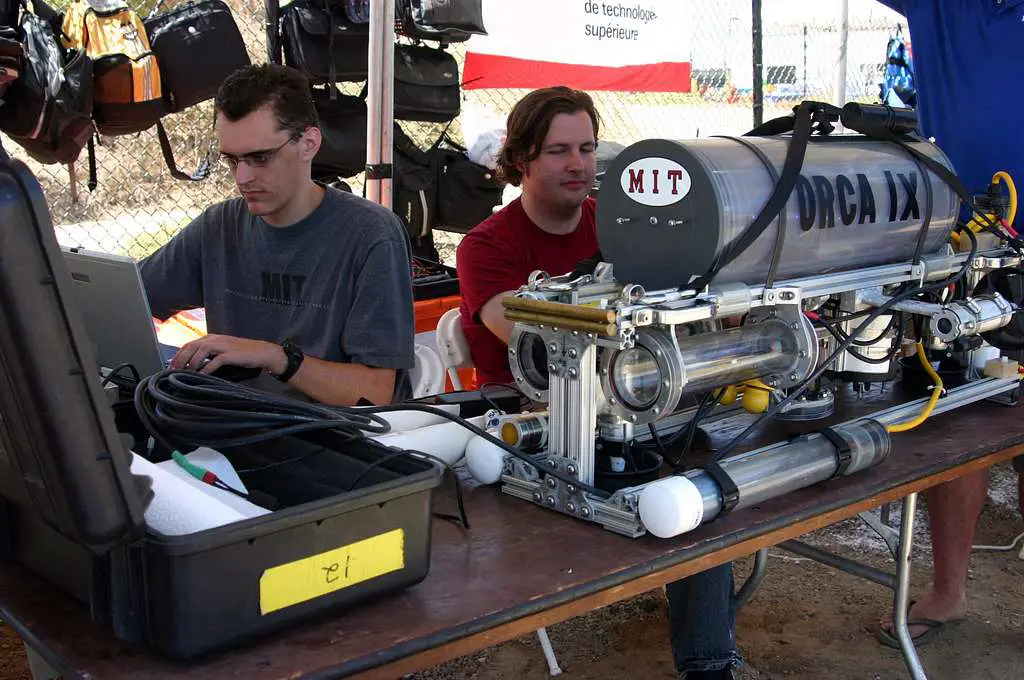
Imagine a drone the size of a penny with muscles instead of motors. MIT’s team developed low-voltage dielectric elastomer actuators—think artificial muscles—that flap tiny wings at 120 Hz, boosting payload by 80% while slashing voltage by 75%. According to TechXplore, these “soft muscles” are built from 20 alternating layers of elastomer and ultrathin electrodes, letting the robot hover for record durations.
In the first field tests, the sub-gram flier held steady for over 20 seconds—longer than any prior microrobot—and carried sensors or drug-dispensing cartridges. Unlike rigid machines, its soft actuators absorb collision shock, so it can brush against obstacles without falling apart. The manufacturing trick? A vacuum-aided spin-coating process that minimizes defects in each 10 µm elastomer layer. The result: a robust wing-flexure that endures thousands of flaps. Its tiny body houses four wing modules, each independently driven for pitch, roll, and yaw control. Future iterations could swap in bio-compatible coatings or implantable batteries, letting these bots navigate blood vessels for targeted drug delivery. And because the muscles work at low voltage, on-board electronics become feasible—opening doors to real-time sensing or autonomous decision making. Soft, strong, and sensational.
2. The Hopping Micro-Rover
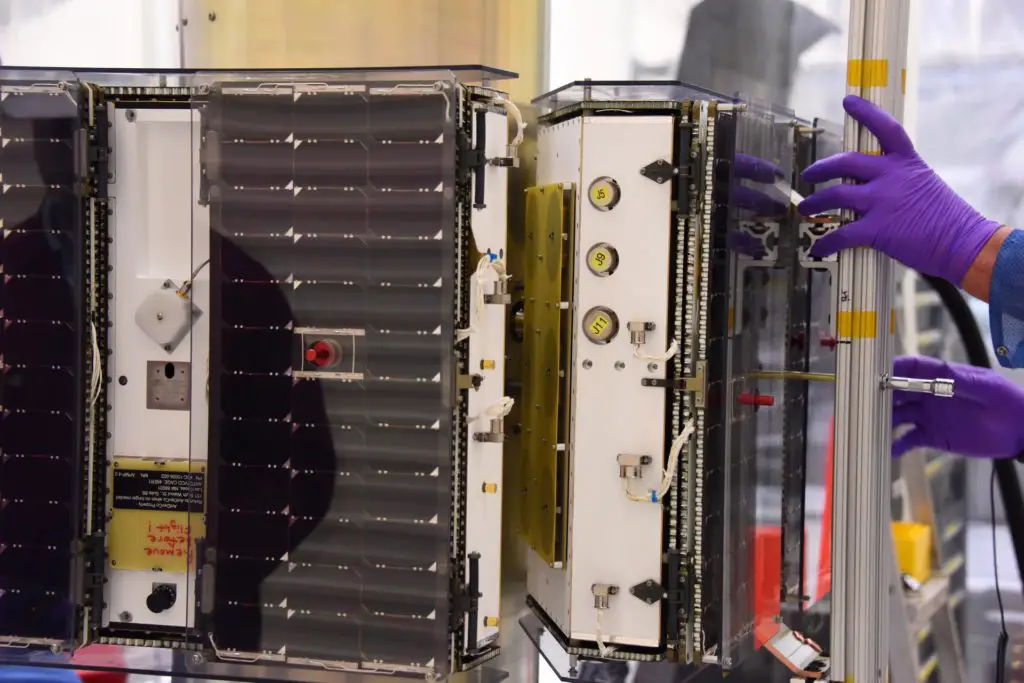
Meet MIT’s hopping robot—a spring-loaded insect-scale explorer that can leap over obstacles and pop back up with less energy than it takes to hover. According to MIT News, it’s smaller than your thumb and weighs less than a paperclip, yet it can clear gaps four times its own height and hop across ice, grass, and even wet soil.
This two-paragraph dynamo combines an elastic “pen-spring” leg with flapping wings that stabilize its arc, so it uses 60% less energy than a pure flyer. In its first demo, it jumped 20 cm at 30 cm/s lateral speed—enough to navigate collapsed buildings or tight pipelines. The extra payload capacity (up to ten times that of a flapping-wing cousin) means you could one day see sensors, batteries, or drug-release modules riding along. Developers envision self-powered versions hopping into disaster zones to map hazards or zipping through the gut to biopsy hard-to-reach lesions. Its controller adapts on-the-fly to surface changes—pumping more wing thrust on slippery patches—so you get seamless transitions from grass to glass. The key insight? Harnessing stored spring energy dramatically boosts endurance. Next up: swapping ground-bounce for aquatic hops, so these bots could inspect underwater fissures too. Tiny, tough, and clever—this little hopper is a major leap for microrobotics.
3. Insect-Scale Pollinators
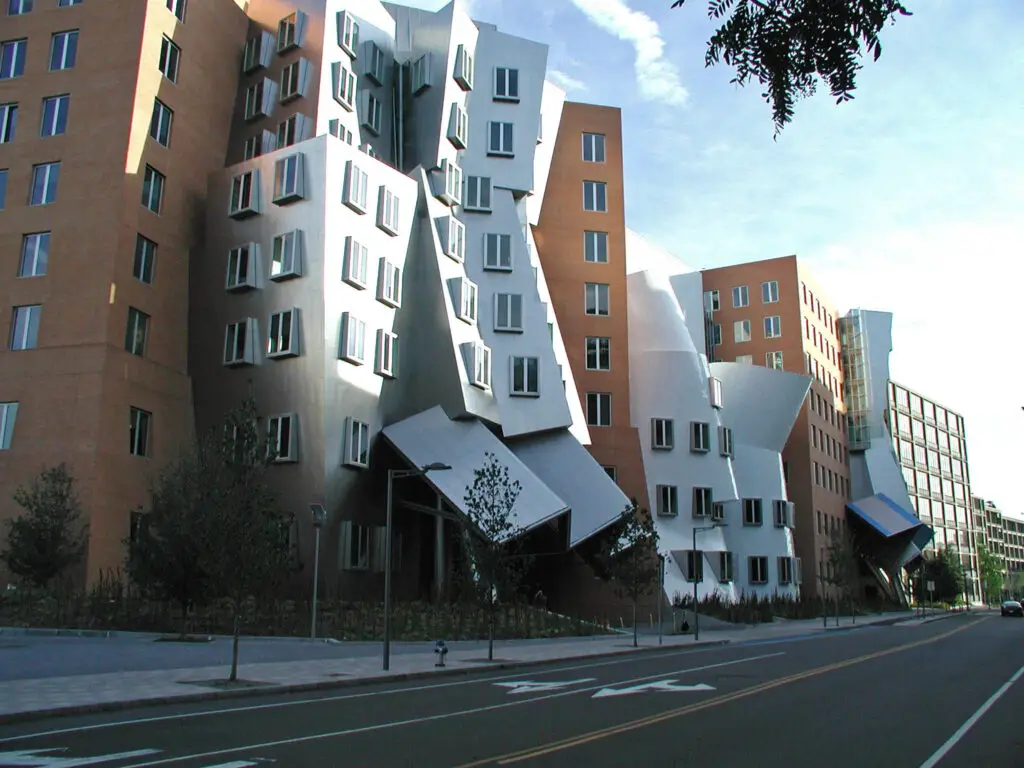
MIT’s “Mechanical Hive” project envisions swarms of robo-insects that buzz into greenhouses to pollinate crops. Inspired by bees, these aerial microrobots demonstrate 100× longer flight endurance than earlier prototypes, flapping reliably for over 1,000 seconds per charge, according to The Debrief. The breakthrough came from optimizing wing flexure geometry and surface-tension-inspired membrane joints.
Each bot weighs under a paperclip and performs acrobatic flips to dodge obstacles, all while carrying pollen-dispensing modules. Researchers tested them in simulated orchard aisles, showing precise flower-to-flower navigation under wireless magnetic guidance. By tuning the wing’s stiffness and wingbeat amplitude, they matched the agility of natural pollinators. Next steps include integrating humidity and fragrance sensors, so the robo-bees can autonomously detect and prioritize unpollinated blooms. Beyond agriculture, similar platforms could carry environmental sensors into hard-to-reach nooks or deliver micro-sprays for targeted therapeutics in human lungs. It’s like Dr. Doolittle meets Dr. Frankenstein—only the flies are machines.
4. Magnetic Millirobot Convoy
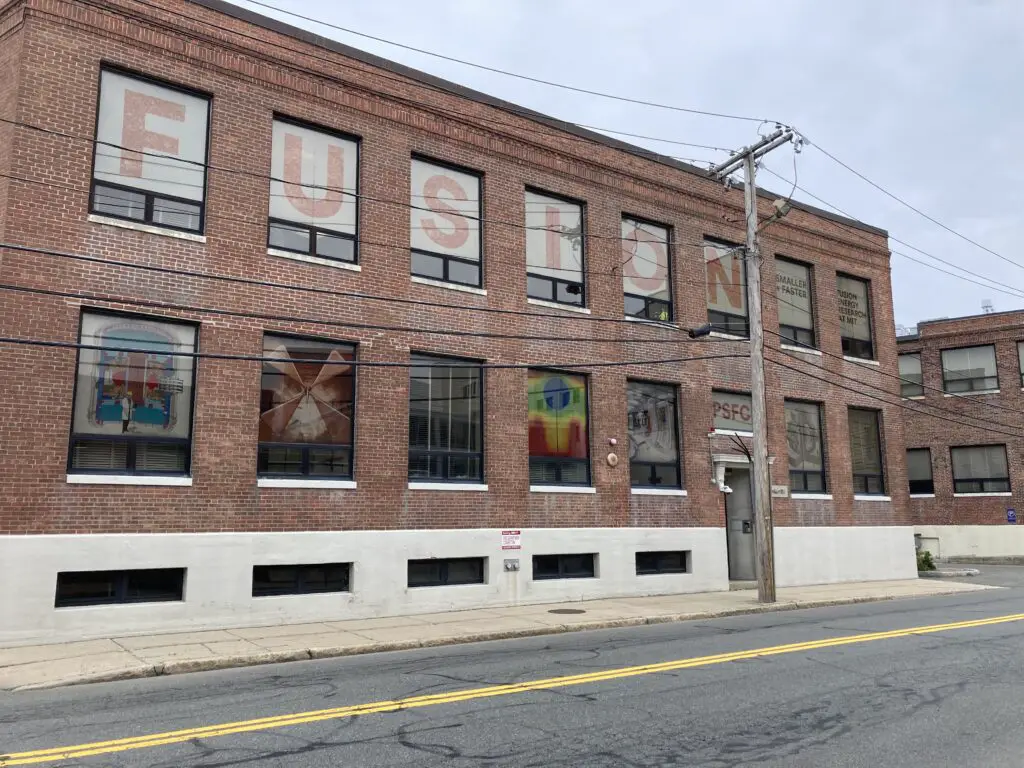
Shrinking robots doesn’t mean ditching teamwork. In a recent study published by a MIT-led team (in collaboration with City University of Hong Kong), magnetically driven millirobots link up like centipedes to haul endoscopic tools through biological lumens, according to arXiv. By forming a convoy, four 5 mm bots generated twice the force of a single unit, enough to carry fluid catheters or electrical wires.
Each unit’s optimized feet grip slippery tissues by alternating adhesive contact—achieving 3× higher traction. A human-scale magnetic array outside the body steers the train through winding intestines, the bile duct, or narrow surgical tunnels. In live–animal tests, the convoy delivered electrocautery instruments to relieve biliary obstructions, then retracted without damaging surrounding tissue. The modular design means surgeons could assemble trains on the fly—literally—by injecting bots and magnetically docking them in vivo. With further miniaturization, these convoys might one day transport micro-biopsies or clear blockages non-invasively. Teamwork makes the dream work, even at the millimeter scale.
5. Biohybrid Jellyfish Injectors
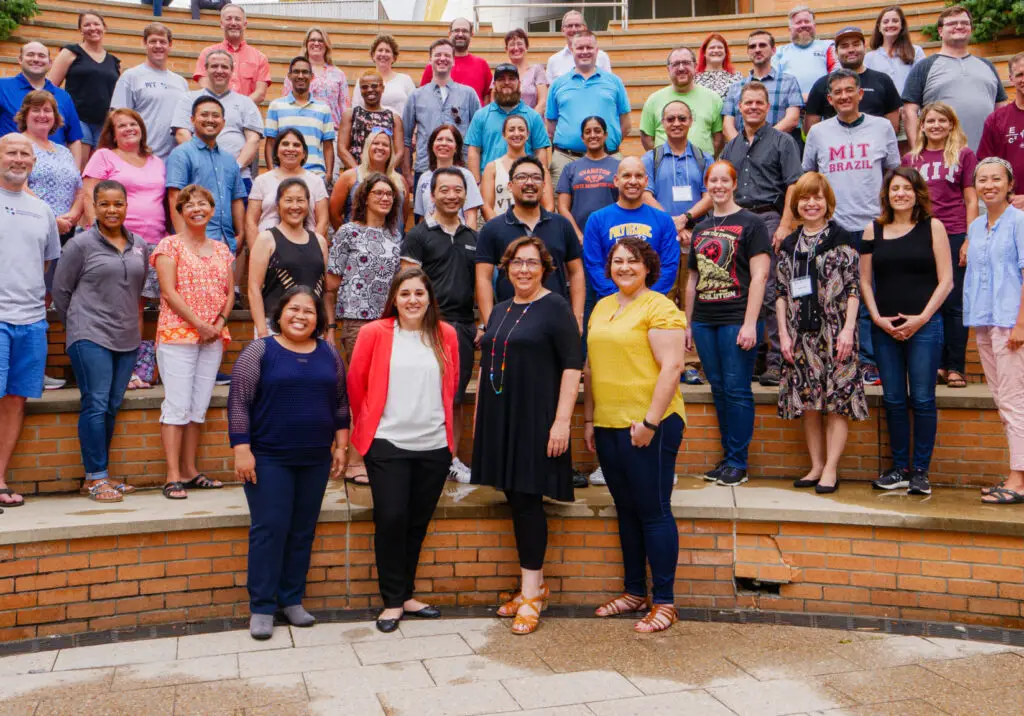
Why stick with metal when you can harness nature? MIT researchers crafted microrobots by grafting jellyfish stinging capsules (nematocysts) onto Janus particles—synthetic beads with one sticky and one slick side. The NIH explains that the biohybrid bots swim to target cancer spheroids, then enzymatically trigger the capsules to eject tubules and release drugs directly into tissues.
Externally controlled by magnetic fields, the Janus particles roll toward tumors with precision, while the jellyfish components act as nanoinjectors—penetrating deep into tissue without piercing blood vessels. In vitro trials with C. elegans and 3D tumor spheroids showed efficient delivery and minimal off-target leakage. Because the capsules only activate upon enzyme exposure, the system ensures payload release only at diseased sites. Next versions could load different nematocyst types for versatile cargo—DNA, mRNA, or high-potency toxins—while retaining biocompatibility. This marriage of biology and robotics points the way to smart, self-powered drug micromanufacturing inside the body.
6. Endoscopic Soft Capsule Robot
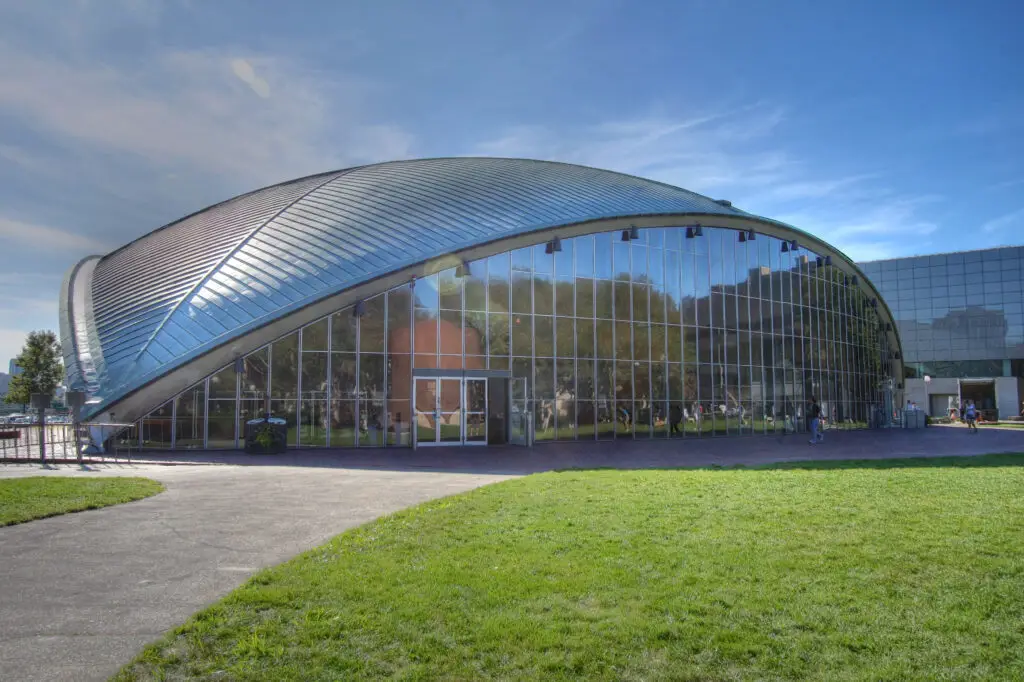
Swallowable soft millirobots—think high-tech Pez dispensers—slide through the GI tract, capturing tumors or performing biopsies en route. These pill-shaped bots inflate micro-fins to crawl along mucus, then deploy micro-cutting tools to resect tissue samples. Their soft polymer skins adapt to curved organs, reducing discomfort and perforation risk. Researchers envision real-time ultrasound feedback guiding these bots, enabling gastroenterologists to diagnose early-stage cancers without sedation.
And the best part? These little capsules can be programmed to park in a specific spot—say, next to a suspicious lesion—then wait for a remote “go” signal before springing into action. Battery-free versions harvest energy from stomach acid, so you don’t need to worry about running out of juice mid-journey. Once they’ve done their job, they deflate and pass naturally, leaving no metal scraps behind. Down the line, imagine integrating tiny cameras or pH sensors to monitor ulcer healing over days, all without a single invasive procedure. It’s like sending a mini-Doc McStuffins to patrol your insides, only way cooler—and cheaper—than traditional endoscopies.
7. Acoustic Nanorobotic Communicators
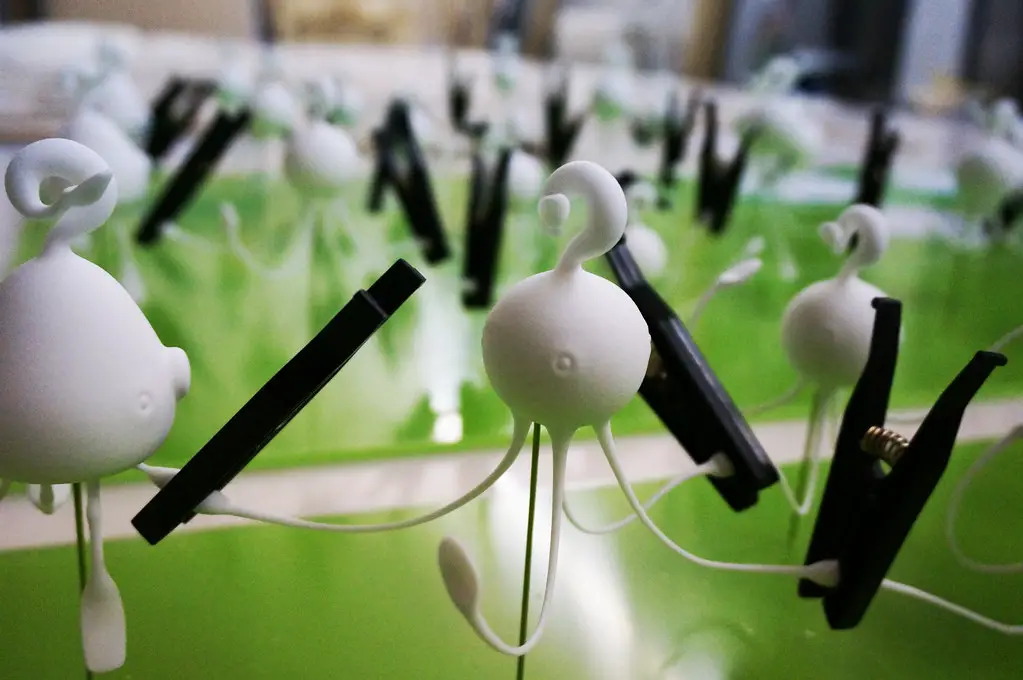
At the nanoscale, radio waves are useless, so MIT scientists demonstrated ultrasonic communication protocols for swarms of nanorobots. Using directional acoustic beams at 100 MHz, they achieved 10 kbps data rates across 100 µm distances with negligible tissue damage. With this network, trillions of circulating nanobots could coordinate to form 3D imaging arrays or orchestrate synchronized drug release pulses—opening up programmable in vivo diagnostics.
Think of it as Morse code, but for microscopic machines whispering in ultrasound. Each nanobot can act as both transmitter and receiver, creating a mesh that self-heals if a few members go offline. Because ultrasound penetrates deep into tissue, these bots stay in touch even in the darkest corners of your body—no Wi-Fi required. Future upgrades might enable them to triangulate their positions for real-time 3D maps of blood flow or tumor boundaries. And with built-in error-correction algorithms, they won’t get their wires crossed when telling each other to “release payload now.” It’s the ultimate squad-chat for robo-cells.
8. Zinc-Air Battery-Powered “Digital Employees”
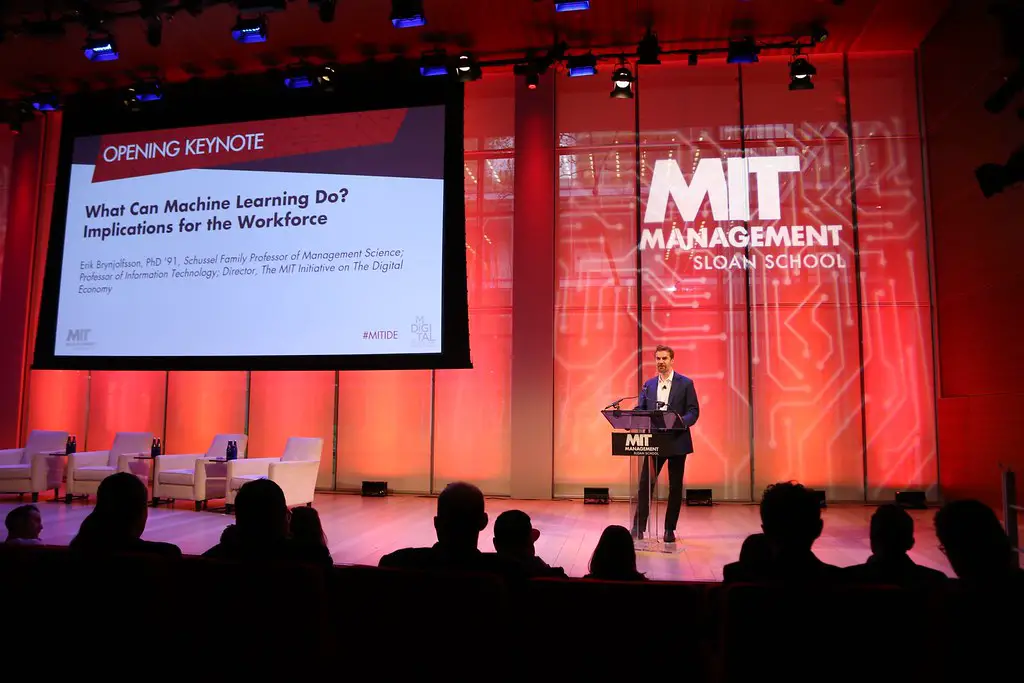
Smaller than a grain of sand, zinc-air cell–powered microbots promise continuous operation in bodily fluids. MIT engineers paired sand-sized zinc anodes with oxygen from the environment to sustain autonomous swarms for hours. Applications range from targeted vascular repairs to leak detection in industrial pipelines. Imagine fleets of these bots patrolling arteries, patching early aneurysms before they burst.
But wait—there’s more geeky magic: these zinc-air cells can be recharged in situ by simply switching the local chemistry or exposing them to light-activated catalysts. That means a morning dose of microbots could handle your entire day’s diagnostics, then recharge overnight inside you like little robotic fireflies. The trick lies in a nanoporous air electrode that balances oxygen intake and reaction speed, preventing bubble buildup that would slow things down. With future tweaks, these bots could even scavenge metabolic waste as an extra fuel source—talk about green energy! Plus, since zinc and oxygen are biocompatible, the system sidesteps nasty heavy-metal toxicity issues. Your body becomes its own micro-charging station.
9. Worm-Inspired Soft Scalloper
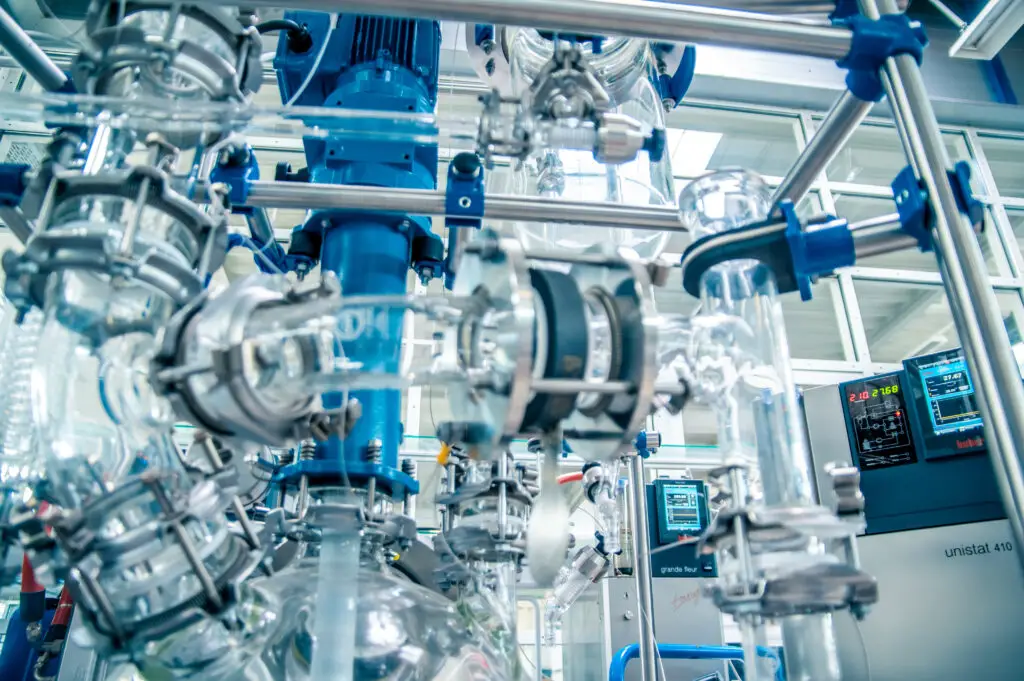
Inspired by earthworms, MIT’s soft ‘scalloper’ microrobot expands and contracts to inch through narrow capillaries. Its peristaltic motion, powered by shape-memory alloys, exerts gentle pressure on vessel walls, propelling pulsed drug gels along its body. Early tests in microfluidic channels show accurate timing of release zones, enabling spatial choreography of multi-drug cocktails—ideal for staggered chemotherapy regimens.
Beyond drug delivery, the scalloper’s gentle crawling could survey vessel health by measuring real-time resistance and elasticity. Each expansion cycle doubles as a micro-pressure gauge, feeding data to an external console for physicians to spot early signs of vascular stiffening. Need to navigate a bend? The scalloper’s segmented design lets it pivot almost like a tiny inchworm doing yoga, hugging curves without scraping the lining. And because it moves so smoothly, there’s minimal inflammatory response—key for long-term implants. Picture a future where a scalloper caravan scouts your arteries daily, delivering drugs only where and when they’re needed. It’s slow and steady that wins the health race.
10. Magnetic Tumor-Tracing Swirlers
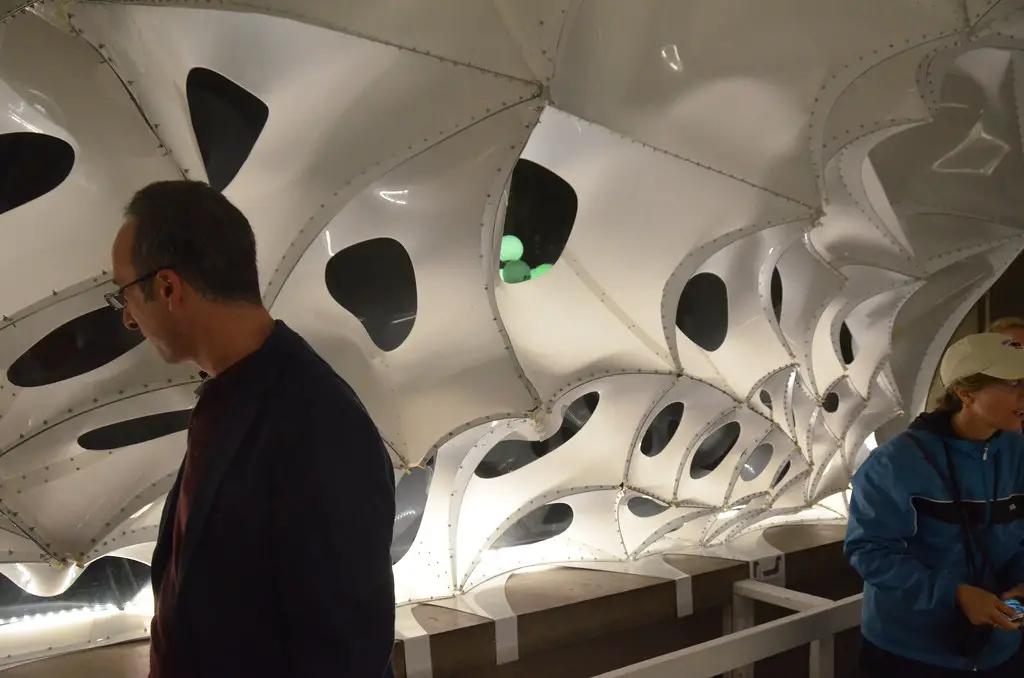
Harnessing rotating magnetic fields, researchers devised microscale spirals that drill into tumor masses. By combining magnetostatic selection fields and precise rotation control, these bots overcome dense extracellular matrices, paving channels for co-injected therapeutics. In mouse models, swirlers improved nanoparticle penetration by 50%, boosting chemo efficacy with half the dose.
But here’s where it gets really trippy: swirlers can team up in formation, creating microscopic “drill rigs” that bore deeper into solid tumors while carrying sensors to map tissue density. Each spiral’s tip is coated with enzyme-loaded nanogels that slowly digest stroma, clearing a path for its buddies. Once the highway is carved, drug-laden nanoparticles flood in unimpeded—think VIP express lanes to tumor cells. And if you tweak the magnetic field’s frequency, you can tune how fast each swirler spins, fine-adjusting penetration depth on the fly. Tumor-busting micro-drills, now with added precision drilling.
11. Micro-Swimmer Sperm Bots
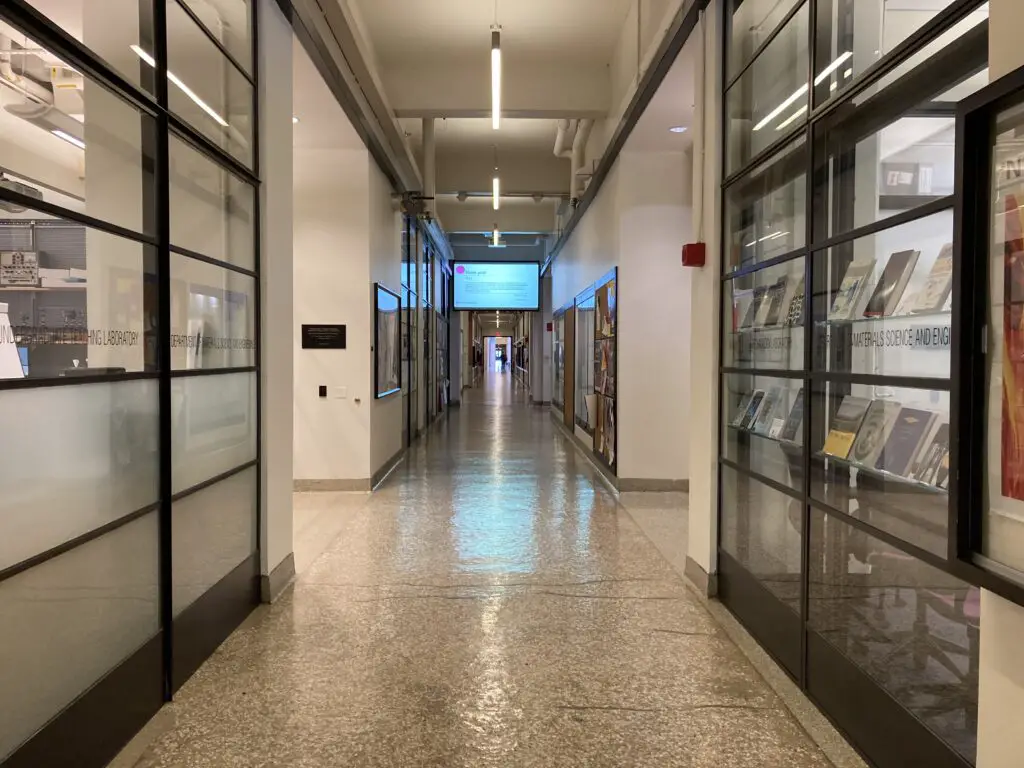
Borrowing biology again, MIT’s team attached drug-laden nanoparticles to immotile spermatozoa to create “spermbots” for reproductive tract delivery. Guided magnetically, these biohybrids reach the oviduct to deposit fertility drugs or perform microsurgery—potentially treating ectopic pregnancies or enabling non-invasive IVF.
But these aren’t your average swimmers: each spermbot carries a tiny onboard gyroscope—a nano-gyro—that keeps it pointed in the right direction against fluid currents. Once it arrives, built-in pH sensors can tell it to release its cargo only when it hits the precise microenvironment of the fallopian tube. And because sperm membranes fuse naturally with epithelial cells, the transfer of drugs or genetic material is seamless and efficient. Future versions could even harvest energy from the sperm’s own flagellar motion, making them self-powered until they finish their mission. It’s reproductive tech meets nanorobotics in a dance floor that’s microscopic but high-stakes.
12. Nanofabricated Compliant Springs
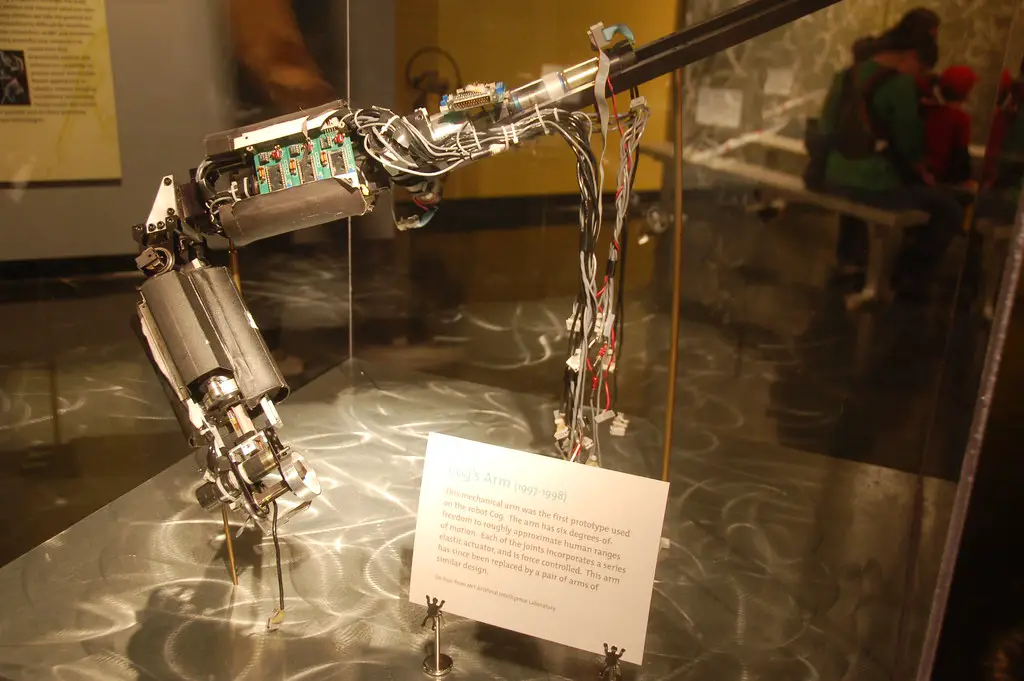
At the nanoscale, MIT’s 3D-printed acrylic-elastomer springs measure forces as low as 0.5 pN. Integrated into microrobots, these springs provide tactile feedback, enabling bots to gauge tissue stiffness and modulate drug release in fibrotic versus healthy regions—essential for personalized therapy.
And because these springs are printed with sub-100 nm resolution, you can program each coil’s geometry to tweak stiffness profiles across a single device. That means one microrobot could carry multiple springs, each tuned to a different force threshold—say, one to sense a tumor boundary and another to judge healthy muscle density. When the spring compresses, it drives a nano-valve that opens or closes drug channels, creating a feedback loop between touch and treatment. Researchers are even testing “spring arrays” that act like tiny fingertips, mapping mechanical properties in 3D as they traverse tissues. In essence, these bots don’t just deliver drugs—they feel their way to the sweet spot.